Understanding the Process and Steps in DNA Replication
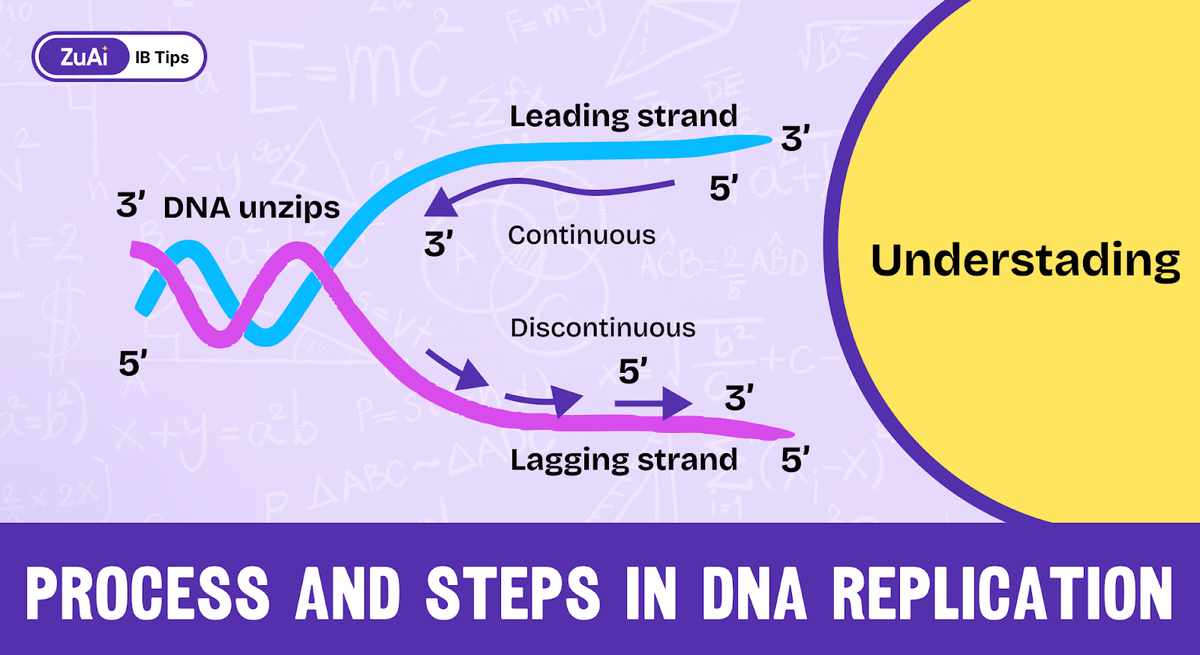
Mastering biological processes like DNA replication can feel overwhelming, especially when preparing for high-stakes IB exams. Research has shown that human cells replicate their DNA with an astonishing accuracy of about 1 error per billion nucleotides, highlighting the precision and complexity of this process.
DNA replication is not just another topic in biology—it’s the cornerstone of understanding how life sustains itself. This process ensures that genetic material is accurately duplicated during cell division, enabling organisms to grow, heal, and pass traits to offspring. For students, grasping the DNA replication steps is essential for excelling in exams and appreciating the intricacies of cellular functions.
In this blog, we’ll break down the steps of DNA replication into clear, actionable insights. Let’s dive in and explore solutions.
Also read: How to Score a 5 on AP Exams: Tips and Study Techniques for College Board Success
What is DNA Replication?
DNA replication is a highly organized and precise biological process where a single DNA molecule is duplicated to produce two identical copies. This process takes place before a cell divides, ensuring that each new cell receives a complete set of genetic instructions. It is central to the continuity of life, as it allows organisms to grow, repair damaged tissues, and reproduce by passing on genetic material.
The process involves a sequence of steps that unwind the DNA’s double helix, separate its strands, and use each as a template to create complementary strands. This mechanism is vital for maintaining the integrity of genetic information across generations. Whether it occurs in simple prokaryotic cells or complex eukaryotic cells, the core principles of DNA replication remain the same.
Why is DNA Replication Important?
DNA replication underpins essential cellular functions like growth and repair. Without accurate replication, cells would be unable to divide properly, leading to errors in genetic material that could disrupt vital processes or even cause disease.
During cell division, replication ensures that every daughter cell inherits an identical copy of the DNA. This precise duplication safeguards the organism’s genetic blueprint, preserving the instructions required for creating proteins, enzymes, and other molecules critical for life. For multicellular organisms, DNA replication also enables complex processes like development and reproduction, ensuring the transfer of traits from one generation to the next.
In short, DNA replication is the foundation of genetic stability and continuity, making it a cornerstone of both cellular biology and the study of life itself.
Initiation of DNA Replication
The initiation of DNA replication is a critical phase where the groundwork for duplicating the DNA molecule is laid. This process occurs at specific sequences on the DNA molecule called origins of replication, which act as starting points. The efficiency and accuracy of replication depend on the precise orchestration of several molecular events that prepare the DNA strands for copying. Let’s break these events down:
Unwinding the DNA Double Helix
At the origin of replication, an enzyme called DNA helicase attaches to the DNA molecule. Its primary function is to unwind the tightly coiled DNA double helix, breaking the hydrogen bonds between complementary base pairs. This separation exposes the two single strands, which will serve as templates for synthesizing new DNA.
The unwinding creates a Y-shaped structure known as the replication fork, where the replication machinery operates. Each replication fork consists of two single strands moving apart as helicase continues to unwind the helix. This step is crucial for providing access to the DNA’s nucleotide sequences, enabling the synthesis of new strands.
Stabilizing the Strands
Once the DNA strands are separated, they tend to rejoin due to the attraction between complementary base pairs. To prevent this, single-strand binding proteins (SSBs) attach to the exposed single strands. These proteins stabilize the strands by keeping them apart and protecting them from enzymatic degradation.
Stabilization ensures that the replication machinery, including enzymes like DNA polymerase, can access the template strands without interruptions. This step is essential for maintaining the integrity of the replication process.
Enzyme Activation
Specific proteins and enzymes come into play during initiation to assemble the replication machinery. These initiation proteins bind to the origin of replication, ensuring that the complex of enzymes and helper molecules is correctly assembled. This replication complex includes helicase, primase, and DNA polymerase, among others.
The coordinated activation of these enzymes ensures that the replication process proceeds efficiently and accurately. In prokaryotes, the initiation is simpler due to a single origin of replication, while in eukaryotes, the presence of multiple origins on long DNA molecules requires more complex regulation.
With the DNA strands prepared and stabilized, the next phase involves synthesizing new DNA strands—a process as intricate as it is essential.
Steps of DNA Replication
DNA replication is a well-organized, step-by-step process that ensures accurate duplication of genetic material. Each step contributes to the seamless creation of two identical DNA molecules. The process involves three primary stages:
Unwinding the Double Helix
The first step in DNA replication is unwinding the tightly coiled double helix. This is achieved by the enzyme DNA helicase, which binds to the origin of replication and breaks the hydrogen bonds between complementary base pairs (adenine with thymine and cytosine with guanine).
As the bonds break, the two strands of the DNA molecule separate, exposing their sequences. This creates a Y-shaped structure called the replication fork, where the DNA strands are ready to serve as templates for the synthesis of new complementary strands. This unwinding process also generates tension in the DNA molecule, which is alleviated by the action of topoisomerase, an enzyme that prevents supercoiling and stabilizes the fork.
Priming the Template Strands
Once the DNA strands are unwound, they require preparation for synthesis. An enzyme called primase creates short RNA primers on the exposed template strands. These primers are necessary because DNA polymerase, the enzyme responsible for building new DNA, cannot initiate synthesis on its own.
The RNA primers provide a starting point for DNA polymerase to attach and begin adding nucleotides. Each primer is complementary to the DNA template it attaches to, ensuring accuracy in the replication process. This step is crucial for initiating DNA synthesis on both the leading and lagging strands.
Synthesizing New DNA Strands
The actual construction of new DNA strands occurs in this step. DNA polymerase binds to the RNA primer and starts adding nucleotides to the growing DNA chain. It synthesizes the new strand by following the base-pairing rules: adenine (A) pairs with thymine (T), and cytosine (C) pairs with guanine (G).
- On the leading strand, DNA polymerase synthesizes continuously in the same direction as the replication fork.
- On the lagging strand, synthesis occurs in short, discontinuous segments called Okazaki fragments, as the strand runs in the opposite direction. These fragments are later joined by DNA ligase to form a complete strand.
DNA polymerase not only adds nucleotides but also proofreads the newly synthesized strands to correct any errors, ensuring high fidelity in the replication process.
With the double helix unwound, primers in place, and new strands synthesized, the next step involves understanding how continuous and discontinuous replication differs on the DNA strands.
Continuous and Discontinuous Replication
DNA replication is a bidirectional process, meaning that replication occurs simultaneously on both strands of the DNA molecule. However, because the two strands run in opposite directions (antiparallel orientation), replication proceeds differently on each strand. This leads to two distinct modes of synthesis: continuous replication on the leading strand and discontinuous replication on the lagging strand.
Leading Strand: Continuous Replication
The leading strand is synthesized continuously in the same direction as the replication fork's movement. DNA polymerase, the enzyme responsible for adding nucleotides to the growing DNA chain, can work uninterrupted as helicase unwinds the double helix.
- Mechanism:DNA polymerase attaches to the primer on the leading strand and begins adding nucleotides in the 5’ to 3’ direction, which aligns with the opening of the replication fork. This allows for a smooth, linear extension of the new DNA strand without pauses.
- Advantages:Continuous replication on the leading strand ensures rapid and efficient synthesis of one-half of the DNA molecule.
This straightforward process demonstrates how the orientation of DNA strands influences replication, making the leading strand simpler to replicate compared to its counterpart.
Lagging Strand: Discontinuous Replication
The lagging strand, oriented in the opposite direction, poses a challenge for DNA polymerase. Since the enzyme can only add nucleotides in the 5’ to 3’ direction, it cannot synthesize the lagging strand continuously. Instead, replication occurs in short, discontinuous fragments known as Okazaki fragments.
- Mechanism: As helicase unwinds the DNA, short stretches of the lagging strand become accessible. Primase lays down RNA primers on these sections, providing starting points for DNA polymerase. The enzyme then synthesizes short fragments of DNA.Once a fragment is completed, DNA polymerase detaches and moves to the next available primer, repeating the process. The gaps between Okazaki fragments are later sealed by DNA ligase, forming a continuous strand.
- Challenges:The stop-and-go nature of discontinuous replication makes this process more complex and slower than leading strand synthesis.
Key Differences Between Leading and Lagging Strands
Students often struggle to understand the complexity of lagging strand synthesis. ZuAI helps students simplify complex topics like lagging strand synthesis with AI-powered flashcards and mock exams, making challenging concepts easier to understand.
The distinction between continuous and discontinuous replication highlights the intricacy and precision of DNA replication, ensuring that the entire DNA molecule is faithfully duplicated despite its structural challenges.
Roles of Enzymes in DNA Replication
DNA replication is a highly coordinated and intricate process that involves several enzymes working together to ensure the accurate copying of genetic material. These enzymes play distinct, yet interdependent roles, allowing the cell to duplicate its DNA efficiently and with high fidelity. Below are the key enzymes involved:
DNA Polymerase
DNA polymerase is the enzyme responsible for adding new nucleotides to the growing DNA strand during replication. It reads the template strand and synthesizes a complementary strand by matching each nucleotide with its corresponding base pair. This enzyme ensures the integrity of the new DNA strand by proofreading as it works, correcting any errors in base pairing. Its proofreading ability significantly reduces the chances of mutations, maintaining the accuracy of the genetic code.
In addition to its role in elongation, DNA polymerase also plays a crucial part in the replication of the lagging strand, where it works in conjunction with other enzymes to form Okazaki fragments.
DNA Ligase
DNA ligase is essential for linking the Okazaki fragments, which are small sections of DNA synthesized on the lagging strand. Since DNA replication is discontinuous on this strand, the process generates numerous short fragments that need to be joined together. DNA ligase seals the "nicks" between these fragments, creating a continuous DNA strand. Without DNA ligase, these gaps would remain, and the replication process would be incomplete, compromising the integrity of the new DNA strand.
Topoisomerase
During DNA replication, the DNA double helix is unwound by helicase, creating tension ahead of the replication fork. This tension can lead to excessive twisting or supercoiling, which can hinder the replication process. Topoisomerase alleviates this issue by making temporary cuts in the DNA molecule to relieve the strain, preventing the DNA from becoming overwound. Once the tension is reduced, topoisomerase reseals the cuts, ensuring the replication fork remains stable and can continue unwinding the DNA. This action is vital to maintain the efficiency and stability of the replication process.
These enzymes work in concert, each playing a critical role in the DNA replication machinery. Their cooperative efforts allow the cell to duplicate its genetic material with remarkable precision and speed, ensuring that the genetic information is faithfully transmitted to the next generation of cells.
Once the new DNA strands are synthesized, the replication process moves toward completion.
Termination of DNA Replication
The termination of DNA replication marks the final stage of this complex process, ensuring that the entire genome has been accurately copied. While the core mechanisms are similar across prokaryotes and eukaryotes, there are key differences in how the replication process concludes, depending on whether the organism has circular or linear DNA. Here's how termination happens in both:
Replication Forks Meet (Prokaryotic Cells)
In prokaryotic organisms, such as E. coli, DNA replication begins at a single origin of replication and proceeds bidirectionally, forming two replication forks that move in opposite directions along the circular DNA molecule. These forks eventually meet at a specific location on the DNA, marking the end of the replication process.
When the replication forks converge, the two newly synthesized strands join together, completing the replication of the entire circular DNA molecule. This ensures that the entire genome has been copied, and the process terminates. The convergence of the replication forks essentially seals the newly synthesized DNA into two complete, identical circular chromosomes.
Telomerase Completes Synthesis (Eukaryotic Cells)
Eukaryotic cells face a more complex challenge during DNA replication due to their linear chromosomes. The ends of these chromosomes, known as telomeres, cannot be fully replicated by standard DNA replication machinery because the DNA polymerase enzyme cannot fully replicate the very ends of the chromosomes (due to the "end replication problem"). As a result, without special mechanisms, the telomeres would progressively shorten with each round of cell division, leading to the potential loss of important genetic information.
To solve this, eukaryotic cells rely on the enzyme telomerase. Telomerase adds repetitive nucleotide sequences to the ends of the telomeres, extending them and allowing for the complete replication of the chromosome ends. This addition of telomeric DNA prevents the loss of critical genetic information and ensures that the chromosomes remain intact over multiple generations of cell division. Telomerase is particularly active in cells that undergo frequent division, such as germ cells, stem cells, and certain cancer cells.
In some cells, telomere shortening can contribute to aging and cell death, making telomerase an important enzyme in regulating cellular longevity.
Final Steps in Termination
Once telomerase has extended the telomeres in eukaryotic cells, and the replication forks have met in prokaryotes, the termination phase is complete. Any final nicks or gaps in the DNA are sealed by DNA ligase, ensuring that the newly replicated DNA is structurally intact. For prokaryotes, the circular DNA is now fully replicated, while for eukaryotes, each linear chromosome has been completed, and the telomeres are protected from further erosion.
With the termination of DNA replication, the cell now has two identical sets of DNA, ready to be divided between daughter cells during cell division.
These final steps ensure that the entire genome is accurately duplicated and ready for distribution to daughter cells during cell division.
Also read: The Impact Of AI Tools In Education: Next Level of Classroom Learning
Conclusion
DNA replication is a precise and essential process that ensures your cells function properly and your genetic information is accurately passed on. Understanding this process is crucial for both students studying for exams and anyone interested in the wonders of biology.
At ZuAI, we understand how important it is to grasp complex concepts with ease and confidence. Our personalized learning tools are designed to support you every step of the way, offering tailored resources to help you succeed. Whether you're preparing for exams or simply deepening your understanding, we’re here to help you achieve your goals.
Contact us today to learn how ZuAI can support your learning journey and help you approach your studies with clarity and confidence. We’re here to assist you in mastering even the most challenging topics.
FAQS
1. How do cells deal with errors during DNA replication?
Cells have repair mechanisms like mismatch repair and proofreading by DNA polymerase to correct errors during DNA replication, preventing mutations.
2. Why do Okazaki fragments form on the lagging strand?
Okazaki fragments form because DNA replication occurs in the 5’ to 3’ direction, requiring the lagging strand to be synthesized in short pieces, which are later joined.
3. What happens if telomerase is not active during DNA replication?
If telomerase is not active, the telomeres shorten with each cell division, which can lead to cell aging or death. This process is linked to aging and some diseases.
4. What is the significance of the replication bubble in DNA replication?
The replication bubble forms when DNA unwinds at the origin of replication. It allows for the simultaneous copying of both DNA strands, speeding up the replication process.
5. How does the speed of DNA replication vary between organisms?
DNA replication occurs at different speeds in different organisms. For example, prokaryotic cells replicate faster than eukaryotic cells due to their simpler structure and circular DNA.